Roadmap to Sustainable Printed Electronics | TNO at Holst Centre
- khashayar Ghaffarzadeh
- Sep 18, 2023
- 9 min read
Updated: Sep 19, 2023
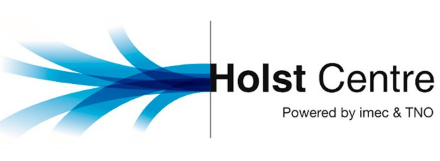
Stephan Harkema and Corné Rentrop | TNO at Holst Centre | stephan.harkema@tno.nl
A growing desire for continuous data collection, real-time information, and connectivity has resulted in increased demand for electronic functionalities that are fully integrated in everyday objects. Consumer electronics, healthcare, wearable electronics, IoT, and smart packaging are examples of market segments that follow this trend. Printed circuit boards (PCBs) are state-of-the-art when it comes to creating electronic functions, but pose challenges with respect to sustainability. These highly integrated electronic products, where plastics, metals and semiconductor components are seamlessly combined, are a challenge to recycle. The only suitable end-of-life scenario is still limited to shredding and incineration. With increasing electrification, digitization, broad wireless integration (IoT) and welfare, the amount of waste from electronics and electronic devices increases drastically. An estimated 1.2 Mtons of Printed Circuit Boards (PCBs) end up in the total amount of annual e-waste[1]. Only a third is recycled in environmentally sound facilities. This means that around 800 million kilograms may be traded, recycled in a non-compliant and polluting manner or may end up on a landfill. There is a more eco-friendly alternative to PCBs: Hybrid and Printed Electronics (HPE). This technology is a big step forward compared to PCBs, but much more is needed to achieve circular production of next-generation electronics.
Despite their name, printed circuit boards (PCBs) are not actually printed. They consist of multiple layers of electroplated copper on glass-fiber epoxy substrates onto which electrical components, so-called surface-mounted devices (SMDs) are assembled. The copper layers are etched with hazardous chemicals to create the circuitry. At end-of-life, the encapsulated copper and unrecyclable epoxy carrier make PCBs a recycling challenge. Transitioning from PCBs to HPE (Hybrid and Printed Electronicprovides immediate opportunities to achieve ecological benefits: reduced device thickness, lower weight, printed sensors, and additive instead of deductive manufacturing of the circuitry. Printed electronics is a disruptive technology that is on the verge of breakthrough in the automotive, lighting, and medical domains. Each use case represents a slimmer, lighter, more appealing, more practical, and less polluting alternative to the conventional device based on printed circuit boards (PCBs). However, in both types of electronics, PCBs, and HPE, the circuitry and SMDs contain potentially critical, high-impact metals embedded in plastics to protect them from external influences, such as mechanical damage and moisture. As opposed to PCBs, the environmental impact of HPE can be further reduced by using recyclable, bio-based, and renewable or compostable materials.
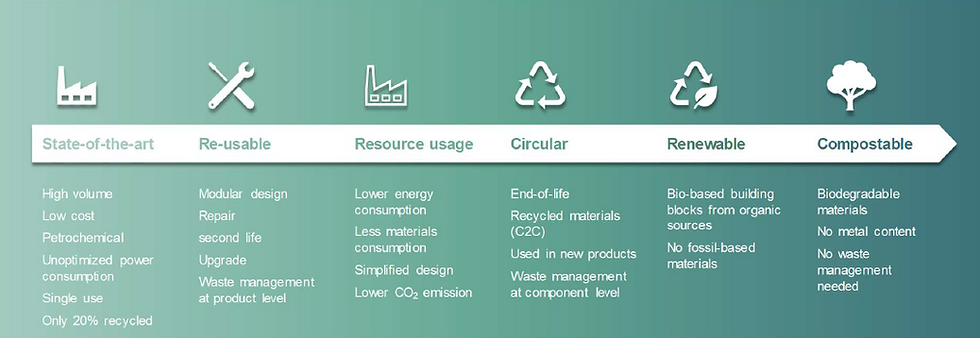
Figure 1: roadmap to sustainable electronics
Roadmap to sustainable printed electronics
Most, if not all, products are mass produced. A first primary focus lies with a successful market entry which is often achieved by innovative functionalities in an aesthetic product design at competitive price levels. Manufacturing of such products is often not immediately optimized for low material usage, low power usage, and a minimal carbon footprint. Instead, single use and low recyclability are often accepted at this stage to achieve commercial success, which may be essential for the survival probability of the company. Petrochemical materials offer a high degree of reliability, low cost and supply security and are therefore ideally suited for high volume production in this first stage of the roadmap, as shown in Figure 1.
A first step towards increasing a product’s sustainability is to address, and preferably extend, its end-of-life by enabling repairability, for instance by a modular design with repairable or replaceable components, or by enabling upgrades. Such steps do not immediately improve recyclability or reduce the environmental impact of production and the materials, but they do provide a significant improvement to the environmental impact by the simple fact that the product does not need to be replaced by a new one. Also energy consumption during the use phase may be addressed. For some examples, such as devices containing highpower elements (e.g. lighting), optimization of power usage may be an effective means of lowering the overall environmental impact.
Join us at TechBlick's Future of Electronics RESHAPED conference & tradeshow in Berlin on 17-18 OCT 2023 - www.techblick.com/electronicsreshaped. For your spercial attendee discounts please contact us: stephan.harkema@tno.nl ]
Following this second stage, a third step may be taken in which a product undergoes a simplification of its design with a reduction of coatings to limit material usage, production steps and overall energy consumption. Printed electronics technology offers an increasingly credible alternative to PCBs and perfectly matches this stage in the roadmap: 1) lower thickness, 2) printed functionalities instead of discrete SMD components that have a higher footprint, 3) recyclable thermoplastic polymers and 4) additive manufacturing as opposed to deductive etching of copper. Nonetheless, challenges still remain in terms of costs and reliability in comparison to the PCB.
Circular use of materials, as depicted in the fourth stage in Figure 1, requires more drastic changes, especially to enable other choices at end-of-life. When end-of-life is reached, the product needs to be dismantled into its chemical building blocks. Here, printed electronics also provides opportunities, as many of the starting materials are meltable and therefore relatively easy to dismantle. The favoured cradle-to-cradle recycling approach is a possibility to further reduce the environmental impact. However, seamlessly integrated electronic products manufactured to survive rigorous testing in harsh environments cannot be disassembled so easily, which leaves shredding and incineration of these products as the only option at end-of-life. The plastics serve as energy carriers for metallurgic processes. While metallurgic processing at an industrial scale may be very efficient for metal recovery, the plastics are lost entirely. Recuperation of the plastics faces the challenge of achieving a high enough purity level to reach the material properties of the initial plastic. When combining plastics with coatings or metals or other plastics, purity levels plummet and only secondary use is realistic. Recycling of integrated electronics to yield metals, components and plastics separately and in a pure and circularly usable form therefore requires a method of disassembly prior to recycling. Not only does this allow circular use of all components and materials, it also enables higher recycling rates. It is therefore vital for electronics production in a circular economy to include design-for-recycling principles.
In the before-last stage, bio-based materials may be introduced as a replacement for generally used fossil-based polymers. In the final stage, biodegradability is an option for specific use cases where disposal into the environment is likely or a more effective approach to recycling. Devices applied to the human body with a limited lifetime of days to weeks are well-suited to go this far in their sustainable developments, while others intended for long-term use are less likely to benefit from biodegradability. Materials from bio-based origins are considered to be environmentally friendly, but large volume production may put additional strain on food supplies and animal feed (1st generation feedstock) or land use for non-food biomass (2nd generation)[2]. Algae are a promising candidate for third-generation bio-mass due to a very high growth rate (5-10x terrestrial plants)[3]. Moreover, cultivation of algae do not strain food supplies and available cultivated farmland.
Achievable lower environmental impacts
Quantification of the environmental impact typically proceeds through a life-cycle assessment. Guidelines to achieve a lower environmental footprint for printed electronics were provided by Prenzel et al.[4]. Copper has a significantly lower global warming potential than silver, possibly up to a factor of 70[5]. While this choice for a different metal would lead to a more than 95% lower contribution to the GWP, it is limited to the printed circuitry alone, if processing remains otherwise identical. Similar values are possible for the
plastic carrier if e.g. paper is a suitable alternative. However, reliability would then be at stake due to a high sensitivity to moisture/water, revealing the potential, but also the intricacies related to developing sustainable electronic products. At a higher integration level, ultra-thin organic photovoltaic solar cells were reported to demonstrate a 10-85% reduction in carbon footprint by a combination of a different substrate, less silver and a modified design[6]. For printed electronics devices closer to industrialisation, a recent life-cycle assessment by Tactotek indicated that, compared to PCBs, in-mould structural electronics (IMSE) enabled a reduction of the carbon footprint by 34-62%, depending on the application and its details[7]. The reductions in carbon footprint for these printed electronics examples are very hopeful and do not stand alone. More examples are available in the literature.
Join us at TechBlick's Future of Electronics RESHAPED conference & tradeshow in Berlin on 17-18 OCT 2023 - www.techblick.com/electronicsreshaped. For your spercial attendee discounts please contact us: stephan.harkema@tno.nl ]
Design-for-recycling (DfR)
The benefits of the application of a design-for-recyclability would further enhance these values as a DfR enables the recovery of the materials. When comparing automated shredding and sorting of PCBs containing electronic waste to manual extraction of the PCB for recycling, higher recovery rates were obtained for the latter (95-99% vs 12-60%)[8]. Another study indicated that extensive dismantling and high-quality recycling would lead to the highest reduction of the product’s global warming potential[9]. Norgren et al. applied design-for-recycling to several use cases and examined the role of a DfR in a circular economy[10]. As described, design-for-recycling holds the potential to increase the quantity and value of materials recovered and reused from products that have reached their end-of-life. Important to realize is that improved recyclability provides a balance between reliability and recyclability: a part is typically designed to withstand rigorous testing (peel tests, pull-out tests, bending tests, stretching, ..), sometimes in combination with harsh environmental exposure (climate chamber set at elevated temperature and humidity, thermal shock tests, aging test at high T and/or with UV exposure). The specs and standards that must be met are often based on conventional PCB electronics and warrant re-evaluation of their applicability in a circular economy. The additional trade-off of recyclability versus costs may come in next, as additional measures or specialistic materials may be necessary to reach the DfR. The question is then when the gains in environmental impact exceed the potential loss in reliability and
or potential increase in costs and which methods for quantification are accepted by the industry and policy makers.
TNO at Holst Centre has developed a DfR approach that can be applied to various printed electronics devices, including in-mould structural electronics, flexible lighting, flexible sensors, and (medical) health patches. In this design, the encapsulant of the printed electronics and discrete semiconductor components can be removed at end-of-life. First demonstrations of the principles on in-mould structural electronics (IMSE) in the EU Treasure project led to successful device disassembly[11]. Upon removal of the polycarbonate encapsulant, that had been applied by injection moulding to a polycarbonate substrate with printed silver circuitry, the printed silver circuitry was exposed. Subsequent hydro-metallurgic processes by Treasure partner Univaq (University of L’Aquila) could target the printed silver directly and specifically without the need to chemically remove the PC beforehand[12]. A full life-cycle assessment, including the comparison of a recycling end-of-life scenario compared to shredding and incineration, is underway.

Figure 2: schematic representation of a design-for-recycling with a) an electronic device encapsulated in plastic, b) first stage of dismantling with the removal of the plastic encapsulant, c) & d) second stage of dismantling that targets SMDs and (printed) circuitry and e) plastic substrate with any leftover (graphic) layers. Figure courtesy of Amires.

Figure 3: a) forced mechanical disassembly of an IMSE device without DfR leading to rupture of the substrate, b) disassembly of an IMSE device with DfR; c,d) Flexible lighting device with DfR in on-state and off-state during peel tests
Join us at TechBlick's Future of Electronics RESHAPED conference & tradeshow in Berlin on 17-18 OCT 2023 - www.techblick.com/electronicsreshaped. For your spercial attendee discounts please contact us: stephan.harkema@tno.nl ]
Acknowledgements
Holst Centre is a research and innovation partner specialized in health technologies, flexible and wireless electronics, powered by the shared expertise of imec and TNO. This research has been funded by the Dutch Ministry of Economic Affairs, and the European Union’s Horizon 2020 research and innovation programme under grant agreement No 101003587 (EU Treasure project), No 101070167 (EU Ecotron project). Original electrical and injection-mould design were realized in the Flexlines project
that received funding from Interreg Vlaanderen-Nederland. The authors would like to gratefully acknowledge the exceptional contributions from Peter Rensing, Pit Teunissen, Jan van Delft, Jeroen Schram, Gerwin Kircher, Adri van der Waal and dr. Margreet de Kok from TNO at Holst Centre, and Sanne Domensino and Joris Vermeijlen from Fontys University of Applied Sciences. We also gratefully acknowledge the wonderful support provided by Rick Leuven and Yibo Su from Brightlands
Material Center for injection moulding with the Flexlines mould.
References:
[1] Baldé, C.P., D’Angelo, E., Luda, V., Deubzer, O., and Keuhr, R. Global Transboundary E-waste Flows Monitor – 2022, United Nations Institute for Training and Research (2022)
[2] Coma M., Martinez-Hernandez E., Abeln, F., Raikova, S., Donnelly, J., Arnot, T.C., Allen, M.J., Hong, D.D., Chuck, C.J.Faraday Discuss., 202, 175 (2017)
[3] Maliha A., Abu-Hijleh, B., Energy Systems (2022) doi:10.1007/s12667-022-00514-7
[4] Prenzel T.M., Gehring, F., Fuhs, F., Albrecht, S. Matériaux & Techniques 109, 506 (2021) doi:
doi.org/10.1051/mattech/2022016
[5] Nuss P, Eckelman MJ (2014) PLoS ONE 9(7): e101298. doi:10.1371/journal.pone.0101298 (2014)
[6] Välimäki, M.K., Sokka, L.I., Peltola, H.B. Int J Adv Manuf Technol 111, 325–339 (2020). doi:10.1007/s00170-020-06029-8 [7] Tactotek webinar “Environmental Performance of IMSE®”, 2022, https://www.tactotek.com/resources/webinar-environmentalperformance-of-imse
[8] Ardente F., Mathieux, F., Recchioni, M. Resources, Conservation and Recycling 92, 158-171 (2014) doi:10.1016/j.resconrec.2014.09.005
[9] Alston, S., Arnold, J. Environ. Sci. Technol. 45, 21, 9386–9392 (2011) doi:10.1021/es2016654
[10] Norgren A., Carpenter A., Heath, G. Journal of Sustainable Metallurgy 6:761–774 (2020). doi: 10.1007/s40831-020-00313-3
[11] Harkema, S., Rensing, P., Domensino S., Verweijlen J., Godoi-Bizarro, D. submitted for publication
[12] Ippolito, N.M.*, Romano, P., Passadoro, M., Pellei, G., Vegliò, F. Treasure Project H2020 - Recycling of automotive waste for the recovery of precious and critical metals: pilot plant tests. The 16th International Conference on Chemical and Process Engineering, Naples, May 21-24, 2023
Comments